Analytical ultracentrifugation (AUC) is a powerful technique for analyzing the physical properties of macromolecules in a solution. Unlike preparative ultracentrifugation, which is primarily focused on isolating macromolecules, analytical ultracentrifuges work by spinning a sample at high speeds while monitoring the movement and distribution of its contents in real-time. AUC is invaluable for biophysics and molecular biology, revealing the size, shape, interactions, and conformational changes of proteins, nucleic acids and nanoparticles. Thus, researchers can evaluate sample homogeneity, binding interactions and aggregation within a sample without labeling or immobilization.
Swedish chemist Theodor Svedberg founded the principles of ultracentrifugation, earning him the Nobel Prize in Chemistry in 1926.1 Advancements in detection devices, data acquisition and analysis tools have significantly improved the applicability of analytical ultracentrifugation in academic and clinical research.
Principles of Analytical Ultracentrifugation
Centrifugation and sedimentation are fundamental principles of AUC. When a sample is subjected to high rotational speeds, the particles within experience a force that pushes them away from the axis of rotation, ultimately settling against the tube's barriers. This process is known as sedimentation.
Conventional centrifuges rely mostly on gravitational force for sedimentation. Due to their small sizes, macromolecules experience negligible gravitational forces. Instead, their movement is mediated primarily by the much greater centrifugal force, a function of the particle mass, angular velocity (rotational speed) and distance from the axis. Centrifugal force is balanced out by buoyant and frictional forces, determining the particle's net sedimentation behavior.
The simplest unit of measure in AUC is the sedimentation coefficient, the rate at which a particle moves in a centrifugal field, which increases with the particle's rotational speed, mass and density. The effect of friction and buoyant forces on a particle is depicted by the frictional coefficient and partial specific volume (i.e., the volume of one gram of particle or solute), respectively.
Types of AUC Experiments and Techniques
Sedimentation Velocity AUC (SV-AUC)
AUC sedimentation velocity experiments aim to measure the sedimentation rate of macromolecules when a centrifugal force is applied. In SV experiments, the solute begins to sediment towards the bottom because of centrifugal forces. On the other hand, frictional and buoyant forces promote the diffusion of solutes. This interplay eventually gives rise to a concentration boundary moving in the direction of sedimentation. Thus, researchers can elicit the dynamic concentration profiles of solutes to study their hydrodynamic properties, molecular mass, shape and size distributions. SV experiments are performed to inspect the solute homogeneity of samples.
Sedimentation Equilibrium AUC (SE-AUC)
In contrast to the sedimentation velocity experiments, sedimentation equilibrium (SE-AUC) works with lower rotor speeds. The sample is spun until the centrifugal, frictional and buoyant forces all reach an equilibrium. Thus, a steady-state concentration gradient of the particle is generated. By repeating the experiment in different solute concentrations, researchers can gain insight into kinetic properties, such as the equilibrium dissociation constants of protein complexes. However, sedimentation equilibrium experiments require longer run times than sedimentation velocity.
Multi-signal and fluorescence-detected AUC
Through advancements in AUC detection methods, researchers can study the heterogeneity of their samples with greater sensitivity and precision. Multiple optical detection systems, such as inference and absorbance optics, can be simultaneously integrated into an analytical ultracentrifuge to track different solutes in the samples. More recently, fluorescence optics became vital to analytical ultracentrifuges, improving their dynamic range and increasing sensitivity to detect solutes found in nanomolar-picomolar concentrations. Integration of fluorescent labeling can help researchers achieve more specific and sensitive analysis with smaller sample volumes required.2
Featured Product
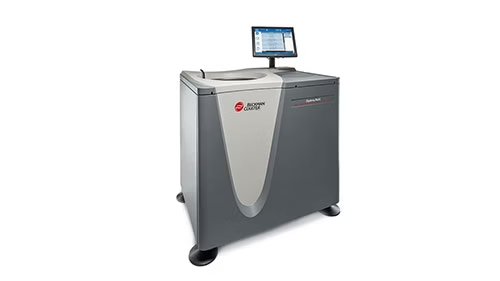
Optima™ AUC
- Enables sample recovery
- Avoids dilution effects
- Analyzes samples in a matrix-free environment
- Has minimal buffer constraints
See how Danaher Life Sciences can help
Data Analysis and Interpretation
Cutting-edge analysis tools are necessary to convert raw signals into quantitative data for interpretation. Therefore, analytical centrifuge experiments must be complemented with software packages.
The forces generated during AUC experiments help create concentration gradients and sedimentation profiles for particles, illustrating the concentration's time-course profile at different radial distances. Data analysis tools can leverage sedimentation profiles to extract key biophysical parameters, such as molecular weight, equilibrium binding constants, shape, elongation and conformational flexibility.
Despite the innovation in data analysis, AUC data analysis presents challenges. Deconvolution of sample content is challenging for highly heterogeneous mixtures, making interpreting sedimentation profiles difficult. Aggregation artifacts can further skew analysis and cause miscalculations of molecular weights or binding constants. Furthermore, analytical centrifuge resolution may not be sufficient to distinguish between species with similar sedimentation profiles.
Applications of Analytical Ultracentrifugation
The molecular characterization prowess of analytical ultracentrifugation is applicable in structural biology, biochemistry and biomedicine.
Protein and nucleic acid characterization
AUC experiments help researchers determine the molecular weights of proteins and nucleic acids while uncovering their monomeric or oligomeric states. Changes in sedimentation profiles can be used to infer conformational changes, such as protein folding.3
Biopharmaceutical and drug discovery research
Analytical ultracentrifugation (AUC) characterizes biopharmaceuticals like recombinant proteins and antibodies. It helps characterize protein-protein interactions and protein-ligand interactions to confirm the bioactivity of therapeutics. Furthermore, AUC can aid in quality control and regulatory compliance by monitoring protein integrity, stability and aggregation.4
Viral and nanoparticle analysis
Developing viral vectors and nanoparticles as drug carriers requires characterization by analytical centrifugation. Sedimentation profiles help determine particle size and integrity, quantify cargo packaging efficiency, and detect impurities in viral vectors, such as host DNA or replication-competent particles.5,6 From this perspective, AUC has a significant role in vaccine and nanotherapeutics development.
Matrix-free separation advantages
Thanks to its matrix-free and solution-based approach, analytical centrifugation provides advantages over separation analysis methods, including chromatography and surface plasmon resonance. Macromolecules can be analyzed without immobilization, which allows monitoring of conformational changes and binding kinetics in native biological conditions.
Challenges and Limitations of AUC
Despite its contribution to macromolecule characterization, analytical ultracentrifugation poses some limitations.
Sample preparation is crucial in AUC workflows. Researchers must follow additional purification steps to ensure the sample is pure enough for AUC analysis. Impurities and minor aggregates can significantly alter results. Furthermore, they must ensure that the solvent of choice is compatible with detection optics.
As previously mentioned, AUC experiments return rich and complex data, which may be overwhelming to interpret. Deconvoluting species with overlapping sedimentation profiles remains a challenge. In addition, optical systems may be inadequate in detecting species with minuscule concentrations or small molecules that settle very slowly. Although fluorescent-based detection significantly improves sensitivity, additional sample preparation steps are required for labeling.
These challenges are partially addressed with AUC automation and AI-driven analysis. Automated workflows improve consistency in sample handling to reduce variability while regulating the temperature. AI-assisted algorithms in software packages can accelerate workflows and enhance accuracy by guiding model selection, parameter estimation, species classification and deconvoluting overlapping species.7
See how Danaher Life Sciences can help
FAQs
What information can be obtained from analytical ultracentrifugation (AUC)?
AUC provides data on molecular weight, size, shape, sedimentation behavior, binding interactions and sample heterogeneity in native solution conditions.
How is AUC used in drug discovery and diagnostics?
AUC assesses protein aggregation, formulation stability and binding affinities, critical for developing safe, effective biopharmaceuticals and diagnostic tools.
How does AUC compare to dynamic light scattering (DLS) or size exclusion chromatography (SEC)?
Unlike DLS and SEC, AUC doesn't require calibration standards or immobilization, offers higher resolution and is more effective at distinguishing between species in mixtures.
Can AUC be used for nanoparticles or non-biological samples?
Yes. AUC can be used to analyze nanoparticles, liposomes, polymers and other colloidal systems, offering insight into size distribution and homogeneity.
How can AUC differentiate between monomers, oligomers and aggregates?
AUC resolves species by their sedimentation coefficients, allowing clear separation and quantification of monomers, oligomers and aggregates in solution.
References
- Svedberg T. The Ultra-Centrifuge and the Study of High-Molecular Compounds. 1937.
- Edwards GB, Muthurajan UM, Bowerman S, Luger K. Analytical ultracentrifugation (AUC): an overview of the application of fluorescence and absorbance AUC to the study of biological macromolecules. Curr Protoc Mol Biol 2020;133(1):e131.
- Basak S, Saikia N, Dougherty L, Guo Z, Wu F, Mindlin F, et al. Probing interdomain linkers and protein supertertiary structure in vitro and in live cells with fluorescent protein resonance energy transfer. J Mol Biol 2021;433(5):166793.
- Bou-Assaf GM, Budyak IL, Brenowitz M, Day ES, Hayes D, Hill J, et al. Best practices for aggregate quantitation of antibody therapeutics by sedimentation velocity analytical ultracentrifugation. J Pharm Sci 2022;111(7):2121-2133.
- Henrickson A, Gorbet GE, Savelyev A, Kim M, Hargreaves J, Schultz SK, et al. Multi-wavelength analytical ultracentrifugation of biopolymer mixtures and interactions. Anal Biochem 2022;652:114728.
- Richter K, Wurm C, Strasser K, Bauer J, Bakou M, VerHeul R, et al. Purity and DNA content of AAV capsids assessed by analytical ultracentrifugation and orthogonal biophysical techniques. Eur J Pharm Biopharm 2023;189:68-83.
- Demeler B. Methods for the design and analysis of analytical ultracentrifugation experiments. Curr Protoc 2024;4(2):e974.
recent-articles